
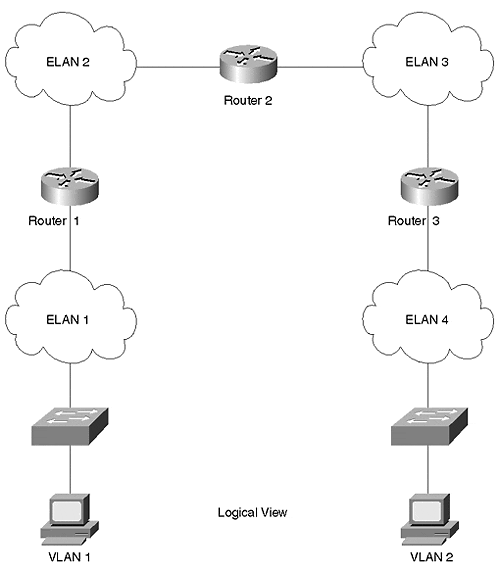
At low concentrations (e.g, Kan 2.5 2.5 μg/ml), and during the early phase of growth (0.5 h), swarm cells were surprisingly more susceptible to killing than planktonic cells, a trend maintained for up to Kan 10 (Supplementary Fig. Exposure to a broad concentration range of the antibiotic kanamycin (Kan) produced distinct survival patterns (Supplementary Fig. Initially, we monitored the antibiotic susceptibility of planktonic vs. Finally, we demonstrate that necrosignaling operates in multiple bacterial species and exhibits species specificity.ĭeath of a subpopulation may be linked to SR Our finding of heterogeneity in cell killing within the swarm is suggestive of altruistic features that ultimately increase survival of the swarm. AcrA binds to the TolC component of this pump on the outer membrane (OM) of live cells to activate immediate efflux, as well as stimulates expression of multiple pathways that overcome antibiotic stress, pathways that are already enhanced in the swarm. We show that the dead cells release a “necrosignal” in the form of AcrA, which is a periplasmic protein component of a tripartite RND efflux pump.
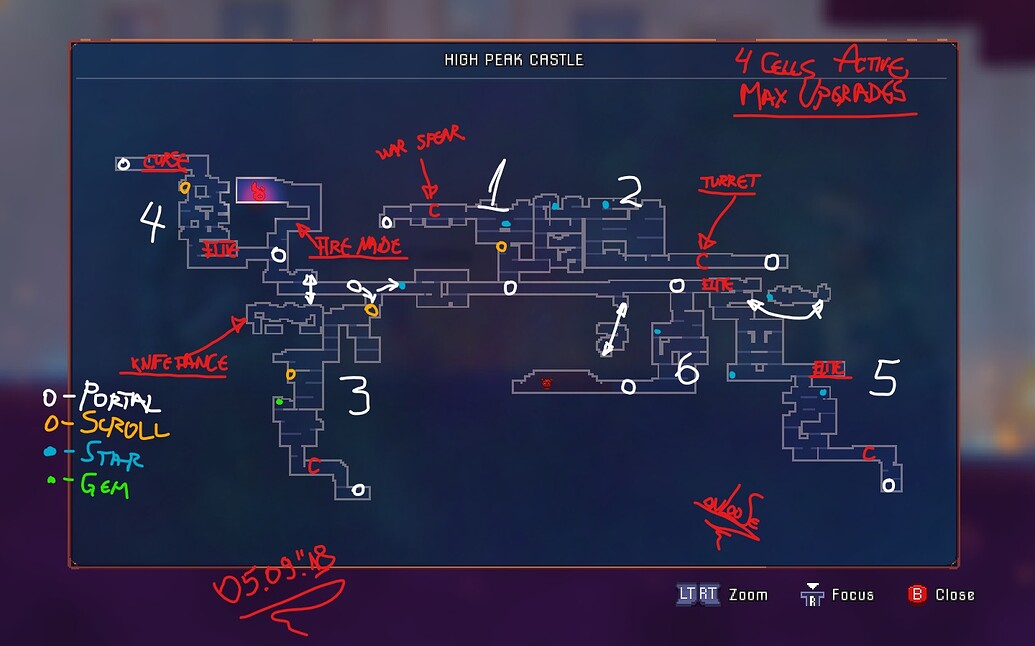
coli), we have used this observation to purify the SR-enhancing factor. In a study focused on Escherichia coli ( E. To test if death of this subpopulation contributes to SR, pre-killed cells were introduced into the swarm, where they indeed enhanced SR. The impetus for the present study came from our observation of heterogeneity in antibiotic-induced cell killing in a swarm, where a distinct subpopulation was more susceptible to killing. Instead, live cells were seen to migrate away from the antibiotic source, leading to a suggestion that cell death emanating around this source releases an avoidance “signal” that contributes to the resistance 20. In a study examining the 3D architecture of a swarm in the presence of different antibiotics, dead cells were not always found at the bottom, ruling out a barrier role 20. Cell death incurred during swarming, however, appeared to provide a protective benefit, leading to speculation that a dead-cell layer at the bottom of the swarm might shield live cells swarming on top by acting as a physical barrier against antibiotic penetration 12. Programmed bacterial cell death, where death of a subpopulation benefits the community by providing nutrients, has been reported by several studies 17, 18, 19. In a study of several bacterial species, swarms that successfully colonized media containing antibiotics nonetheless experienced substantial cell death 12. To distinguish the adaptive resistance seen in swarms from that in other reported cases of this phenomenon, we are calling swarming-specific resistance SR. In stark contrast, bacterial swarms are metabolically active and grow robustly, suggesting that different resistance mechanism/s may be operative here. While several terminologies such as resistance, tolerance, and persistence have been used to describe bacterial resistance phenotypes observed under various settings 14, 15, a shared attribute of a majority these phenotypes is their association with reduced metabolism and slow bacterial growth 16. The resistance depends on high cell densities associated with swarms 12, and is phenomenologically similar to adaptive resistance of bacterial biofilms 13.
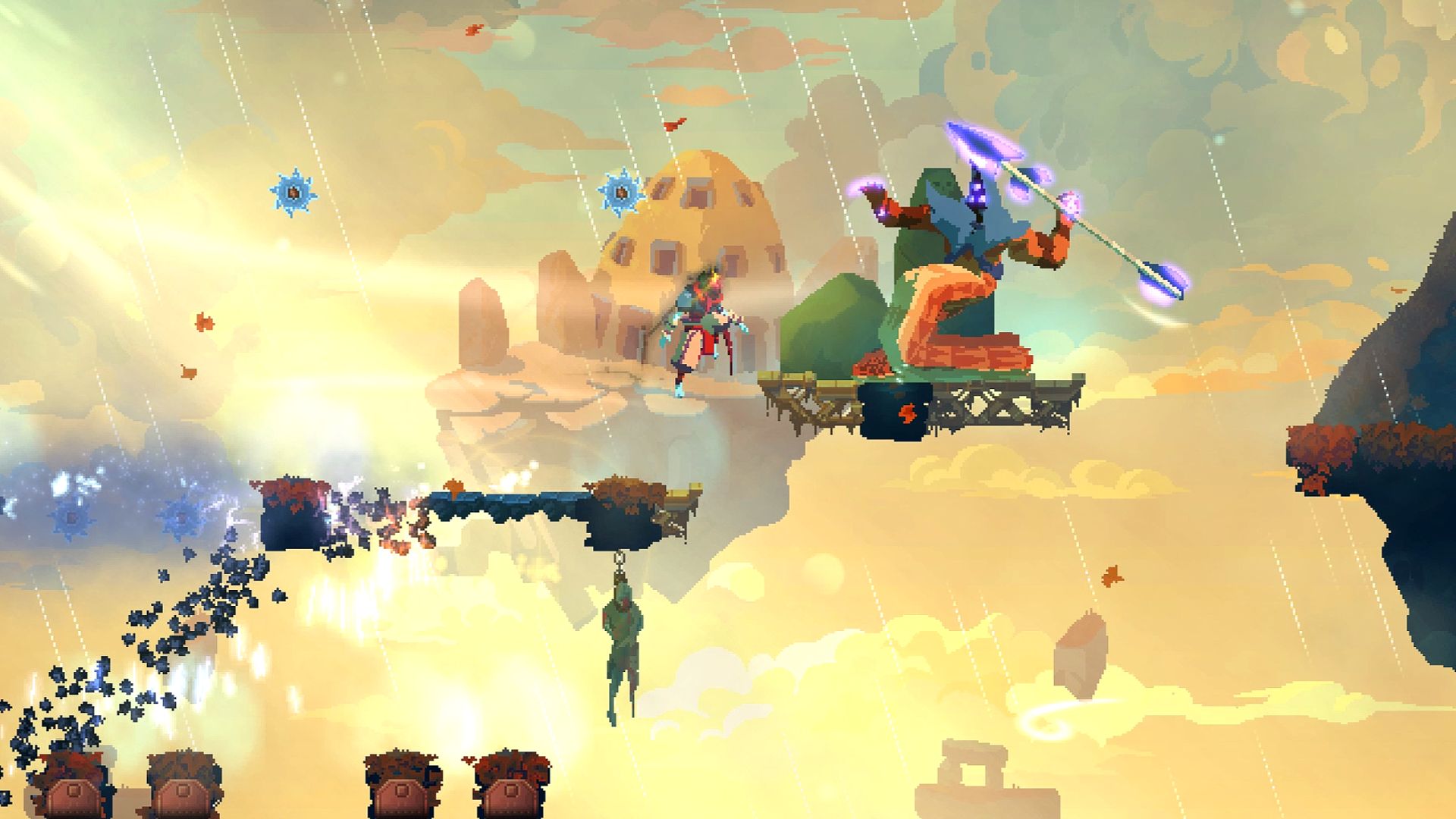
An unexpected and clinically relevant property of swarms is their nongenetic resistance to antibiotics at levels lethal to free-swimming planktonic cells of the same species 8, 9, 10, 11, 12. Flagella are used both for swimming individually through liquid, and swarming collectively over surfaces 3, 6, 7. Of these, flagella-driven motility is the fastest, promoting the colonization of favorable niches in response to environmental signals, and contributing significantly to the pathogenic ability of some species 2, 3, 4, 5. Bacteria employ many appendages for movement and dispersal in their ecological niches 1.
